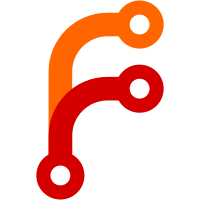
* migrates nav data format and updates docs pages * removes sidebar_title from content files
246 lines
10 KiB
Plaintext
246 lines
10 KiB
Plaintext
---
|
|
layout: docs
|
|
page_title: Transit - Secrets Engines
|
|
description: >-
|
|
The transit secrets engine for Vault encrypts/decrypts data in-transit. It
|
|
doesn't store any secrets.
|
|
---
|
|
|
|
# Transit Secrets Engine
|
|
|
|
The transit secrets engine handles cryptographic functions on data in-transit.
|
|
Vault doesn't store the data sent to the secrets engine. It can also be viewed
|
|
as "cryptography as a service" or "encryption as a service". The transit secrets
|
|
engine can also sign and verify data; generate hashes and HMACs of data; and act
|
|
as a source of random bytes.
|
|
|
|
The primary use case for `transit` is to encrypt data from applications while
|
|
still storing that encrypted data in some primary data store. This relieves the
|
|
burden of proper encryption/decryption from application developers and pushes
|
|
the burden onto the operators of Vault.
|
|
|
|
Key derivation is supported, which allows the same key to be used for multiple
|
|
purposes by deriving a new key based on a user-supplied context value. In this
|
|
mode, convergent encryption can optionally be supported, which allows the same
|
|
input values to produce the same ciphertext.
|
|
|
|
Datakey generation allows processes to request a high-entropy key of a given
|
|
bit length be returned to them, encrypted with the named key. Normally this will
|
|
also return the key in plaintext to allow for immediate use, but this can be
|
|
disabled to accommodate auditing requirements.
|
|
|
|
## Working Set Management
|
|
|
|
The Transit engine supports versioning of keys. Key versions that are earlier
|
|
than a key's specified `min_decryption_version` gets archived, and the rest of
|
|
the key versions belong to the working set. This is a performance consideration
|
|
to keep key loading fast, as well as a security consideration: by disallowing
|
|
decryption of old versions of keys, found ciphertext corresponding to obsolete
|
|
(but sensitive) data can not be decrypted by most users, but in an emergency
|
|
the `min_decryption_version` can be moved back to allow for legitimate
|
|
decryption.
|
|
|
|
Currently this archive is stored in a single storage entry. With some storage
|
|
backends, notably those using Raft or Paxos for HA capabilities, frequent
|
|
rotation may lead to a storage entry size for the archive that is larger than
|
|
the storage backend can handle. For frequent rotation needs, using named keys
|
|
that correspond to time bounds (e.g. five-minute periods floored to the closest
|
|
multiple of five) may provide a good alternative, allowing for several keys to
|
|
be live at once and a deterministic way to decide which key to use at any given
|
|
time.
|
|
|
|
## NIST Rotation Guidance
|
|
|
|
Period rotation of the encryption keys is recommended, even in the absence of
|
|
compromise. For AES-GCM keys, rotation should occur before approximately 2<sup>32</sup>
|
|
encryptions have been performed by a key version, following the guidelines of NIST
|
|
publication 800-38D. It is recommended that operators estimate the
|
|
encryption rate of a key and use that to determine a frequency of rotation
|
|
that prevents the guidance limits from being reached. For example, if one determines
|
|
that the estimated rate is 40 million operations per day, then rotating a key every
|
|
three months is sufficient.
|
|
|
|
## Key Types
|
|
|
|
As of now, the transit secrets engine supports the following key types (all key
|
|
types also generate separate HMAC keys):
|
|
|
|
- `aes128-gcm96`: AES-GCM with a 128-bit AES key and a 96-bit nonce; supports
|
|
encryption, decryption, key derivation, and convergent encryption
|
|
- `aes256-gcm96`: AES-GCM with a 256-bit AES key and a 96-bit nonce; supports
|
|
encryption, decryption, key derivation, and convergent encryption (default)
|
|
- `chacha20-poly1305`: ChaCha20-Poly1305 with a 256-bit key; supports
|
|
encryption, decryption, key derivation, and convergent encryption
|
|
- `ed25519`: Ed25519; supports signing, signature verification, and key
|
|
derivation
|
|
- `ecdsa-p256`: ECDSA using curve P-256; supports signing and signature
|
|
verification
|
|
- `ecdsa-p384`: ECDSA using curve P-384; supports signing and signature
|
|
verification
|
|
- `ecdsa-p521`: ECDSA using curve P-521; supports signing and signature
|
|
verification
|
|
- `rsa-2048`: 2048-bit RSA key; supports encryption, decryption, signing, and
|
|
signature verification
|
|
- `rsa-3072`: 3072-bit RSA key; supports encryption, decryption, signing, and
|
|
signature verification
|
|
- `rsa-4096`: 4096-bit RSA key; supports encryption, decryption, signing, and
|
|
signature verification
|
|
|
|
## Convergent Encryption
|
|
|
|
Convergent encryption is a mode where the same set of plaintext+context always
|
|
result in the same ciphertext. It does this by deriving a key using a key
|
|
derivation function but also by deterministically deriving a nonce. Because
|
|
these properties differ for any combination of plaintext and ciphertext over a
|
|
keyspace the size of 2^256, the risk of nonce reuse is near zero.
|
|
|
|
This has many practical uses. One common usage mode is to allow values to be stored
|
|
encrypted in a database, but with limited lookup/query support, so that rows
|
|
with the same value for a specific field can be returned from a query.
|
|
|
|
To accommodate for any needed upgrades to the algorithm, different versions of
|
|
convergent encryption have historically been supported:
|
|
|
|
- Version 1 required the client to provide their own nonce, which is highly
|
|
flexible but if done incorrectly can be dangerous. This was only in Vault
|
|
0.6.1, and keys using this version cannot be upgraded.
|
|
- Version 2 used an algorithmic approach to deriving the parameters. However,
|
|
the algorithm used was susceptible to offline plaintext-confirmation attacks,
|
|
which could allow attackers to brute force decryption if the plaintext size
|
|
was small. Keys using version 2 can be upgraded by simply performing a rotate
|
|
operation to a new key version; existing values can then be rewrapped against
|
|
the new key version and will use the version 3 algorithm.
|
|
- Version 3 uses a different algorithm designed to be resistant to offline
|
|
plaintext-confirmation attacks. It is similar to AES-SIV in that it uses a
|
|
PRF to generate the nonce from the plaintext.
|
|
|
|
## Setup
|
|
|
|
Most secrets engines must be configured in advance before they can perform their
|
|
functions. These steps are usually completed by an operator or configuration
|
|
management tool.
|
|
|
|
1. Enable the Transit secrets engine:
|
|
|
|
```text
|
|
$ vault secrets enable transit
|
|
Success! Enabled the transit secrets engine at: transit/
|
|
```
|
|
|
|
By default, the secrets engine will mount at the name of the engine. To
|
|
enable the secrets engine at a different path, use the `-path` argument.
|
|
|
|
1. Create a named encryption key:
|
|
|
|
```text
|
|
$ vault write -f transit/keys/my-key
|
|
Success! Data written to: transit/keys/my-key
|
|
```
|
|
|
|
Usually each application has its own encryption key.
|
|
|
|
## Usage
|
|
|
|
After the secrets engine is configured and a user/machine has a Vault token with
|
|
the proper permission, it can use this secrets engine.
|
|
|
|
1. Encrypt some plaintext data using the `/encrypt` endpoint with a named key:
|
|
|
|
**NOTE:** All plaintext data **must be base64-encoded**. The reason for this
|
|
requirement is that Vault does not require that the plaintext is "text". It
|
|
could be a binary file such as a PDF or image. The easiest safe transport
|
|
mechanism for this data as part of a JSON payload is to base64-encode it.
|
|
|
|
```text
|
|
$ vault write transit/encrypt/my-key plaintext=$(base64 <<< "my secret data")
|
|
|
|
Key Value
|
|
--- -----
|
|
ciphertext vault:v1:8SDd3WHDOjf7mq69CyCqYjBXAiQQAVZRkFM13ok481zoCmHnSeDX9vyf7w==
|
|
```
|
|
|
|
The returned ciphertext starts with `vault:v1:`. The first prefix (`vault`)
|
|
identifies that it has been wrapped by Vault. The `v1` indicates the key
|
|
version 1 was used to encrypt the plaintext; therefore, when you rotate
|
|
keys, Vault knows which version to use for decryption. The rest is a base64
|
|
concatenation of the initialization vector (IV) and ciphertext.
|
|
|
|
Note that Vault does not _store_ any of this data. The caller is responsible
|
|
for storing the encrypted ciphertext. When the caller wants the plaintext,
|
|
it must provide the ciphertext back to Vault to decrypt the value.
|
|
|
|
!> Vault HTTP API imposes a maximum request size of 32MB to prevent a denial
|
|
of service attack. This can be tuned per [`listener`
|
|
block](/docs/configuration/listener/tcp) in the Vault server
|
|
configuration.
|
|
|
|
1. Decrypt a piece of data using the `/decrypt` endpoint with a named key:
|
|
|
|
```text
|
|
$ vault write transit/decrypt/my-key ciphertext=vault:v1:8SDd3WHDOjf7mq69CyCqYjBXAiQQAVZRkFM13ok481zoCmHnSeDX9vyf7w==
|
|
|
|
Key Value
|
|
--- -----
|
|
plaintext bXkgc2VjcmV0IGRhdGEK
|
|
```
|
|
|
|
The resulting data is base64-encoded (see the note above for details on
|
|
why). Decode it to get the raw plaintext:
|
|
|
|
```text
|
|
$ base64 --decode <<< "bXkgc2VjcmV0IGRhdGEK"
|
|
my secret data
|
|
```
|
|
|
|
It is also possible to script this decryption using some clever shell
|
|
scripting in one command:
|
|
|
|
```text
|
|
$ vault write -field=plaintext transit/decrypt/my-key ciphertext=... | base64 --decode
|
|
my secret data
|
|
```
|
|
|
|
Using ACLs, it is possible to restrict using the transit secrets engine such
|
|
that trusted operators can manage the named keys, and applications can only
|
|
encrypt or decrypt using the named keys they need access to.
|
|
|
|
1. Rotate the underlying encryption key. This will generate a new encryption key
|
|
and add it to the keyring for the named key:
|
|
|
|
```text
|
|
$ vault write -f transit/keys/my-key/rotate
|
|
Success! Data written to: transit/keys/my-key/rotate
|
|
```
|
|
|
|
Future encryptions will use this new key. Old data can still be decrypted
|
|
due to the use of a key ring.
|
|
|
|
1. Upgrade already-encrypted data to a new key. Vault will decrypt the value
|
|
using the appropriate key in the keyring and then encrypted the resulting
|
|
plaintext with the newest key in the keyring.
|
|
|
|
```text
|
|
$ vault write transit/rewrap/my-key ciphertext=vault:v1:8SDd3WHDOjf7mq69CyCqYjBXAiQQAVZRkFM13ok481zoCmHnSeDX9vyf7w==
|
|
|
|
Key Value
|
|
--- -----
|
|
ciphertext vault:v2:0VHTTBb2EyyNYHsa3XiXsvXOQSLKulH+NqS4eRZdtc2TwQCxqJ7PUipvqQ==
|
|
```
|
|
|
|
This process **does not** reveal the plaintext data. As such, a Vault policy
|
|
could grant almost an untrusted process the ability to "rewrap" encrypted
|
|
data, since the process would not be able to get access to the plaintext
|
|
data.
|
|
|
|
## Learn
|
|
|
|
Refer to the [Encryption as a Service: Transit Secrets
|
|
Engine](https://learn.hashicorp.com/vault/encryption-as-a-service/eaas-transit)
|
|
guide for a step-by-step tutorial.
|
|
|
|
## API
|
|
|
|
The Transit secrets engine has a full HTTP API. Please see the
|
|
[Transit secrets engine API](/api/secret/transit) for more
|
|
details.
|